Post
We Already Know
Quite a Bit
20 August 2013
In this series we started with the basic observations of stellar motion in a galaxy, and how that led to the idea of some kind of dark matter. We saw that modified gravity models couldn’t explain things like the Bullet Cluster, while dark matter could. We also saw that dark matter must be something other than the normal matter that makes up you and me. It must be non-baryonic, weakly interacting with light, and make up a goodly portion of galaxies. Then again, our best candidate for dark matter is a hypothetical particle based on a particle physics model that is quite probably wrong.
So why do scientists keep talking about dark matter like it’s real? Isn’t this all just a dodge of simply covering up “we don’t know” with the name dark matter so we can get research grants from the government?
No.
In this series I’ve roughly followed a historical approach to dark matter, starting with the first evidence pointing in the direction of dark matter and showing how alternatives to dark matter are ruled out. But in science the real convincing evidence is not simply one matching example, or one prediction that wins the day. What really brings a model to the level of established scientific theory is that it matches observation over and over again. When new types of data come in, they also match the model (which is basically a prediction). Of course this isn’t done with great astronomical images, it’s done with data and graphs, which is much less sexy than pretty pictures.
So how does the dark matter model match up with observation? Extraordinarily well. Consider, for example, the distribution of galaxies in the universe.
Galaxies are not evenly distributed throughout the universe. Instead they clump together into clusters separated by large voids. The amount of clumping depends on the amount of dark matter in the universe. If there is more dark matter in the universe, the galaxies clump more tightly, and if there is less (or no) dark matter then it would clump less. It is not just the amount of clumping, but the scale at which clumps form. Do galaxies clump into lots of little clusters that are evenly distributed, or do small clusters clump into larger, superclusters?
At the cosmic scale, this clumping is driven only by mass and the gravitational interactions of that mass. We’ve measured the position of galaxies going out to 2 billion light years in various directions, so we basically have a 4 billion light-year wide chunk of the universe where we can compare the distribution of galaxies to different models.
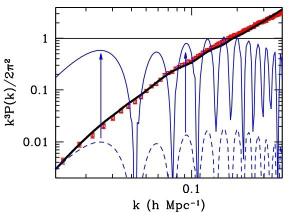
One way to look at the clumping distribution is known as a power spectrum. Basically just take the total average density of the observed galaxies, then you split the sky into two regions and take an average of each section, then split and average again, and so on. Keep doing that, and you get an “average” density at each scale. The result can be seen in the figure here.
The red data points represent the actual power spectrum for the universe out to two billion light years. The dotted blue line represents the power spectrum you would get in a universe of Einstein’s gravity and no dark matter. The solid blue line is the power spectrum you would get with the relativistic MoND model. That is, the “modified gravity” approach. The solid dark line is the expected power spectrum for a universe with dark matter. Which one do you think best matches the data?
This graph more than all the other evidence I’ve mentioned should convince you that dark matter is real. It accurately describes the universe we observe over 4 billion light years. It is so accurate that it actually distinguishes a specific dark matter model known as ΛCDM. The CDM stands for cold dark matter, and the Λ or “lambda” represents the cosmological constant (dark energy).
By itself this would be overwhelming evidence in support of dark matter, but we can also look at the fluctuations in the cosmic microwave background, and make a power spectrum of its “clumpy-ness”. I’ve written about this before. This also fits the same model.
Dark matter is real. From the power spectrum observations of galaxy distributions and the cosmic microwave background, as well as other observations, we not only know dark matter exists, we know that the total mass of dark matter is about 6 times that of regular matter. We have a good understanding of the average density of dark matter in the universe. We know all this from large amounts of data that agrees with the model, and keeps agreeing with the model.
But what about the idea that the dark matter particle is a WIMP known as the neutralino? As I mentioned last time, the neutralino is a hypothetical particle derived from supersymmetry. The latest findings from the Large Hadron Collider seem to indicate that supersymmetry (at least in its basic form) doesn’t work. Aren’t we back to having no ideas about dark matter?
Not quite. In the supersymmetry model, predictions for the mass of the lightest neutralino are on the order of 100 times the mass of a proton or more. Given the density of dark matter in the universe, there would be lots of these dark matter neutralinos passing through the Earth all the time. If they exist, we should be able to detect them directly.
There have been several experiments trying to do just that. So far we’ve had some interesting possible observations, but nothing with a strong enough result to confirm as an observation. The results from different labs also seem to contradict each other a bit, which is a bit puzzling. Still, if these tentative results are to be believed, they imply that dark matter particles are not 100 times the proton mass, but are closer to 5 to 10 proton masses. This would mean they couldn’t be the neutralinos of supersymmetry.
But this raises an interesting idea, because we know that dark matter mass is about 6 times that of regular matter. Six is between 5 and 10, so if dark matter WIMPs are 6 times the mass of protons, that would imply that there is one dark matter particle for every regular matter (baryonic) one. That type of symmetry is a siren song for theorists, so there is some work being done in that direction. Just to be clear, without solid results this is still a speculative idea, so you shouldn’t take it too seriously just yet.
So that’s where we are at the moment. We know dark matter exists, we know lots about it, and the latest evidence is leading us to consider whole new ideas about not only cosmology but also particle physics.
It’s a very interesting time for astrophysics.
Miss the beginning of the dark matter series? It all starts here.