Post
Ugly Theory,
Beautiful Data
27 October 2012
Yesterday I talked about two approaches to explaining the motion of stars in a galaxy, MOND and dark matter. Today I finish answering Mark Bruce’s question about which model I favor, and why.
When we left our story we had two proposed models: MOND, which posits that for very small forces the acceleration of an object doesn’t quite go to zero, and dark matter, which introduces an invisible “something” that makes up most of a galaxy’s mass. Neither one of these seem particularly appealing, so why (as I stated yesterday) do I favor dark matter as a solution?
Let’s start by looking at MOND again. Yesterday I said its weaknesses were that it was a bit of a kludge, and in its simple form it violates conservation momentum. Both of these are valid criticisms, but neither is enough to just discard the theory. Early quantum theory was also a kludge, and it violated the fundamental tenets of physics. It also happened to be true. MOND is still a young model, so being kludgy is to be expected. There is also the fact that more sophisticated versions of MOND have been proposed that are relativistic, and don’t violate momentum.
That said, any model must make clear predictions, and MOND does. One of the big predictions MOND makes is that for very small forces the acceleration of an object levels off to about 10 trillionths of a gee (1 gee is the gravity we feel on Earth). This is a very tiny force, but in 2007 an experiment was done that tested Newton’s theory down to accelerations 5000 times smaller than the limit proposed by MOND. What they found was that to the limits of their measurements Newton’s theory worked perfectly. Strike one for MOND. This is also a bit of a win for dark matter, which assumes that Newtonian gravity should hold even for small accelerations.
Supporters of MOND have noted that this 2007 experiment looked at inertial forces, not gravitational forces. MOND models usually assume that they apply for all small accelerations, but one could also propose that MOND only applies to gravitational forces (hence the 2007 experiment wouldn’t apply). But if MOND only applies to gravity, then it violates Einstein’s theory of general relativity, which we know works very well. Again, this isn’t a deal-breaker, but it does mean more kludgy fiddling with the model to make it work.
So now let’s look at dark matter a bit. Proposing invisible undiscovered particles to fix your problem is a dangerous path to tread, but let’s look at what dark matter predicts. The 2007 experiment is nice to have, but it isn’t really a prediction. It would be better to have a prediction we can test. This can be found through an effect called gravitational lensing.
In general relativity, gravity is described as a curvature of space and time. Matter warps space around it, which produces the appearance of a force we call gravity. This warping of space also means that light near a mass will be deflected slightly. As a result, light passing near a galaxy is focused by the galaxy’s mass, similar to the way a lens focuses light. We’ve observed this effect around lots of galaxies. The more mass a galaxy has, the more the light will be deflected, so we can use gravitational lens measurements to determine the mass of a galaxy.
This leads us to a dark matter prediction. If dark matter really does exist, and it makes up most of the mass of a galaxy, then the mass measured by gravitational lensing should be much higher than the visible mass we see in a galaxy. This is in fact exactly what we find. The visible matter in a galaxy seems to be a small fraction of the total mass. Mark this as a win for dark matter.
Our initial MOND model predicted that the mass should be mostly visible. But our first experiment required that MOND apply only to gravity (and violate general relativity). If that is true then MOND might also deflect light, which would give the appearance of extra mass that isn’t really there. MOND can still be made to work. But continually tweaking a theory to match new data isn’t the way science works. Having your model agree with past observation is necessary, but not sufficient. Ideally your model should make a solid prediction before the outcome is known.
So at this point we have two experimental findings. Dark matter agrees with the first and predicted the second. MOND made the wrong prediction for the first, didn’t predict the second, but can be modified to agree with both. Dark matter seems to be winning, but it still predicts a new type of “something” that 1) isn’t regular matter 2) hasn’t been observed. As the saying goes, extraordinary claims require extraordinary evidence. Just because it works better than the alternative doesn’t mean we should accept dark matter.
It would be nice if we could have a prediction that is unexpected. Preferably one that contradicted MOND. It turns out we have just such a thing in colliding galaxies.
Let’s assume for a moment that dark matter exists. This would mean that two approaching galaxies would have both regular matter and dark matter. As the galaxies collide, the regular matter (stars, gas, dust) interact with each other, which slows them down. But the dark matter would only weakly interact with matter, so it shouldn’t slow down. Instead the dark matter from the two galaxies should pass through each other and keep going. This means that in a galactic collision the regular matter should bunch up in the middle while the dark matter passes through to the other side. If that is true, then the distribution of visible mass should be different than the distribution of dark matter as measured by gravitational lensing. This is a radical idea, and it completely contradicts MOND. According to MOND the distribution of visible mass should agree with the distribution seen by gravitational lensing.
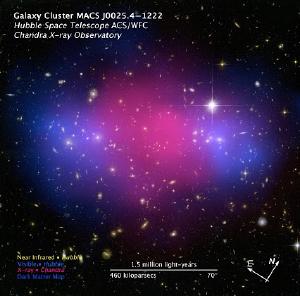
For this experiment, dark matter wins big time. The first colliding galaxies where the two distributions were compared is known as the Bullet Cluster. The results were published in 2004, and they showed a clear difference between the visible mass and lensing mass distributions. In 2008 another pair of colliding galaxies (known as MACS J0025.4) showed the same difference. You can see this one in the figure. The red shows the distribution of visible mass, while the blue shows the mass distribution seen by gravitational lensing. MOND predicts the two should overlap. Dark matter predicts that it should be red in the center and blue on either side. Which result do you see?
So what have we found? Out of three experimental results, dark matter agreed with the first outcome and predicted the other two correctly. MOND on the other hand incorrectly predicted the first and third. It seems like every time we get new data, MOND has to go back to the drawing board for revisions. A scientific model lives or dies based on the evidence, and the evidence doesn’t look good for MOND.
This is why I’m in the dark matter camp. It has made accurate predictions, and it agrees with observation without wild tweaking. It also agrees with our understanding of cosmology. When we make computer models of cosmic evolution, the ones with dark matter agree with observation, while the ones without don’t. So dark matter is actually on a pretty strong footing, which is why most astrophysicists are in the dark matter camp.
Of course dark matter still has a serious challenge. It predicts a new kind of matter that we haven’t seen in the lab. It would be nice to make a direct detection of dark matter. This is actually an active area of research. So far there are some interesting hints, but nothing conclusive.
In the end, the data will have the final say.