Post
Two By Two
20 September 2013
Yesterday I talked about the detection of gamma ray bursts, intense blasts of gamma rays that occasionally appear in distant galaxies. Gamma ray bursts were only detected when gamma ray satellites were put into orbit in the 1960s. This is because gamma rays are absorbed by our atmosphere. Even then, the detectors were relatively primitive and couldn’t determine the direction of the bursts. Instead, multiple satellites were used to triangulate the location of these bursts. Since then, gamma ray astronomy has gotten much more sophisticated.
Gamma ray photons are so energetic that observing them requires tools from particle physics. As we’ve gotten better at particle physics detectors, we’ve gotten better gamma ray telescopes. You can see this in the Fermi Gamma-ray Space Telescope, launched in 2008.
You may remember that x-ray telescopes require long focal lengths, and therefore are typically the size of a bus. Since gamma ray photons have even more energy and even shorter wavelengths than x-rays, you might think gamma ray telescopes are huge. But in fact, Fermi is only about 3 meters (10 feet) long, not counting the solar panels. This is because the Fermi telescope is not a telescope in the traditional sense. It doesn’t focus gamma ray light with mirrors or lenses. While it is referred to as a telescope, it is really a particle detector.
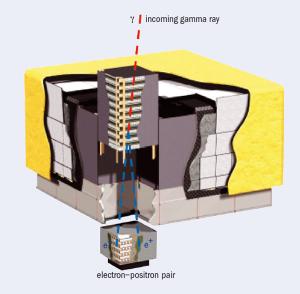
Gamma ray photons have so much energy that when they collide with material they produce a pair of particles, an electron and positron The positron is the antimatter partner of the electron. It is actually this particle pair that is observed by Fermi, as seen in the image. As the gamma ray enters the telescope, it collides with layers of tungsten (known as conversion foils) which causes the gamma ray to produce an electron-positron pair. Layered between the conversion foils are silicon detectors which allow us to determine the direction of original gamma ray. Finally the particle pair strikes a calorimeter, which measures the energy of the particles (and hence the energy of the original gamma ray).
The real challenge is distinguishing gamma rays from high energy cosmic rays. Cosmic rays have an energy similar to gamma rays, but are actually charged particles such as high energy protons or electrons. They too can be observed by the silicon detectors and calorimeter. So to distinguish them, the entire detector is surrounded by another detector known as an anti-coincidence shield. This shield only detects the passing of a charged particle. Since gamma rays are photons (and therefore have no charge) they don’t trigger the shield. Cosmic rays do trigger the shield. So when an event triggers the Fermi detector and the shield you know it is a cosmic ray. When it just triggers the Fermi detector you know it is a gamma ray. In this way you can filter out the signal from the noise.
The real advantage of the Fermi telescope is that it has a wide field of view with a fairly precise resolution. This means over time it can scan the entire sky and know just where gamma rays originate. With it we’ve learned quite a bit about gamma ray bursts. We’ve also learned some other interesting things, but I’ll talk about that next time.