Post
Time After Time
28 March 2013
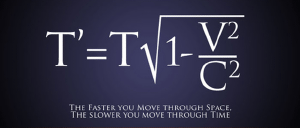
Imagine you are traveling in a train. If you were to walk down the aisle of the train, you would be moving at a walking pace relative to the other passengers, but someone watching the train go by would see you and all the other passengers race by at great speed. In other words, your speed is relative. It depends on what you are measuring it against. Relative to another passenger your speed is slow, but relative to the ground your speed is fast. That, in a nutshell, is relativity.
This concept of relativity dates back at least as far as Galileo (which is why it is sometimes called Galilean relativity). Before Galileo’s time it may have been known, but it wasn’t a big deal because motion could always be measured relative to the fixed Earth. But as we learned the Earth moves around the Sun, this raised an interesting philosophical puzzle. Is there some great cosmic vantage point against which all speeds can be measured, or is it really the case that speed is always relative? Is there such a thing as absolute speed?
In the mid-1800s, physicists came to understand that light was a wave. At the time it was thought that all waves travel through a medium. Sound waves travel through air, water waves travel through water, and so on. That means there must be a medium through which light travels. Physicists couldn’t observe this medium, but they called it the luminiferous (light-bearing) ether. There soon began a hunt to observe the ether, because the ether was a way to measure absolute speed.
If you drop a pebble in a calm lake, you can see the ripples flow outward at a particular speed. The ripples flow with the same speed in every direction. But if you were moving in a boat and dropped a pebble into the water, the ripples would seem to move slower in the direction of the boat’s motion, and faster in the opposite direction. Because of the boat’s motion the speed of the ripples would be different in different directions. The same would be true with the ether. Since the Earth must be moving through the ether, the speed of light must be different in different directions.
In 1887, Albert Michelson and Edward Morley performed an experiment to measure this difference in the speed of light. But what they found was the speed of light was always the same. No matter what direction light travelled, no matter how they oriented their experiment, the speed of light never changed. This was not only surprising, it violated the principle of relativity. After all, if you stand on a moving train and toss a ball, the speed of the ball relative to the ground is the speed of the ball plus the speed of the train, not just the speed of the ball. Basically what Michelson and Morley found was that if your “ball” was light, the speed of your ball relative to the train and the speed of the ball relative to the ground is the same. It seemed the speed of light (and only the speed of light) is absolute, and this made no sense at all.
Then in 1905 Albert Einstein published a solution to the problem, known as special relativity. He demonstrated that if the speed of light is absolute, then time must be relative, as given in the equation above. It relates the different times of two observers, say you and me. In this case, T′ is your time as you measure it, T is your time as I measure it, V is your speed relative to me, and C is the speed of light. What it says is that your time appears slower to me than it does to you. The faster you move relative to me, the slower your time appears to me. This sounds insane. How can time be relative? It is, however, very real.
We can see how this works if we imagine a clock made with light. Take two mirrors and place one above the other and facing each other, then bounce a pulse of light between them. We can measure time by counting the number of times the light bounces off a mirror. Each bounce is like the tick or tock of a mechanical clock. If you could watch the pulse of light, you would see it move up and down between the mirrors at the speed of light. Up and down at a constant rate. Now suppose you took your clock on a fast moving train. Standing in the aisle of the train, you would see the light pulse move up and down at the same rate as before. Up and down at the speed of light.
But as I watch you speed past, I see something slightly different. I would also see the pulse move at the speed of light, but from my view the light can’t move straight up and down because it must also be moving along with you. I would see the pulse move diagonally up then diagonally down, which is a slightly longer distance between each bounce. That means it would take the light longer to travel from bounce to bounce. So from my point of view the ticks and tocks of your clock are slower than the ticks and tocks as you see them. Your clock appears to be running slow because of your motion relative to me. The faster you move relative to me, the more your clock will slow down from my point of view.
You might think this effect only occurs because the clock relied on light to tell time, but this effect is real for everything. If you have a GPS in your phone or car, you rely on relative time being true every time you use it. A GPS determines your location by receiving signals from satellites orbiting the Earth. Those satellites broadcast their time and location, which your GPS uses to determine your position, so it is vitally important that the satellites broadcast the proper time. But the satellites are moving at high speed relative to you, which means their clocks run slightly slow. To give you the accurate time the satellites have to account for that slowdown effect. When your phone tells you where the nearest coffee shop is, it’s using special relativity to do it.
So how does all this relate to astrophysics? It’s one of the ways we know the universe is expanding. When we observe the light from distant galaxies, the light appears more red than we would expect. The more distant the galaxies, the more their light is redshifted. This effect is known as the Doppler effect, and it is due to the fact that the galaxy is moving away from us. The galaxies are moving away from us because the universe is expanding. But suppose over long periods of time light just naturally reddens? How do we know astronomers are not being fooled?
Special relativity tells us we’re not. We can observe supernovae in nearby and distant galaxies, and what we find is that when a supernova goes off in a distant galaxy it happens more slowly than a supernova in a closer galaxy. The time of a distant supernova appears slower to us because the distant galaxy is moving away from us at a faster rate than the closer galaxy.
Strange as it is, special relativity works. Time after time.
Tomorrow: Flying kites in a thunderstorm leads us to a single elegant theory describing lightning, magnets and light. Don’t try this at home, just stay tuned for Part 4.