Post
Einstein and Eddington
19 May 2013
Newton’s laws of motion and gravity predicted the motions of the planets almost perfectly. Newton’s laws are so accurate that we use them to accurately send robotic probes to Mars and other planets, but Newton’s laws aren’t perfect. The motion of some planets differ very slightly from Newton’s predictions. In the case of Uranus, its small deviation led to the discovery of Neptune. In the case of Mercury, however, its small deviation led to a completely new understanding of gravity.
Newton’s law of gravity states that objects are attracted to each other by gravity, and that the amount of attraction decreases the farther apart they are (what is known as an inverse square relationship). As a result of this force, the planets should move around the Sun in elliptical paths. Because of the gravitational pull of the other planets, the motion of each planet isn’t quite a perfect ellipse, but these small deviations can be calculated from Newton’s laws. With most of the planets, the gravitational pull of the other planets accounts for these small deviations.
But this was not the case for Mercury. Mercury is the closest planet to the Sun. It moves so quickly that it makes a complete orbit in about 88 days. As astronomers observed the motion of Mercury, they noticed that it was slowly changing over time. While Mercury essentially followed an elliptical path, just as Newton predicted, its orbit was also slowly changing its orientation. The orbit of Mercury was precessing over time. The rate of precession is very small, only about 43 arc-seconds every hundred years. In other words, the point at which Mercury is closest to the Sun (called its perihelion) was shifting by about 20 miles with each orbit. That might seem like a lot, but it means that Mercury would arrive at perihelion about half a second later than Newton predicted.
Newton’s prediction was only off by about a millionth of a percent, so it is tempting to simply say that is close enough. But in science, when you see a consistent deviation—even a tiny one—it’s worth looking for the cause. Since Newton’s laws were so incredibly accurate, the most likely possibility would be the existence of an undiscovered planet. After all, deviations in the orbit of Uranus had resulted in the discovery of Neptune in 1846.
In 1859, the French mathematician Le Verrier made a detailed analysis of Mercury’s orbit, and hypothesized the existence of a planet even closer to the Sun, which he called Vulcan. Several astronomers made a search for Vulcan, but no such planet was ever discovered. Then in 1915, Albert Einstein proposed a new model of gravity.
A decade earlier, Einstein had developed his theory of special relativity. In special relativity, nothing can travel faster than the speed of light. Time and space are not absolutes, but rather depend upon your motion. Newton assumed that space and time were absolute, and that gravity acted instantly. This contradiction meant that either Einstein or Newton had to be wrong about the way the universe worked.
Einstein started with the assumption that Newton’s gravity must be incorrect. It was a bold assumption given that Newton had worked almost perfectly for nearly 300 years. But Einstein was intrigued by the fact that objects fall at the same rate, regardless of their mass. This idea, known as the principle of equivalence, is why astronauts appear weightless in space. Gravity is still pulling on them, but because everything (including their spaceship and everything in it) falls at the same rate, the astronauts have the illusion of being weightless. Because of the principle of equivalence, an astronaut in a windowless spaceship wouldn’t be able to tell if she was in deep space where there is no gravity, or if she was simply falling in orbit around the Earth. Both cases would give the same feeling of weightlessness.
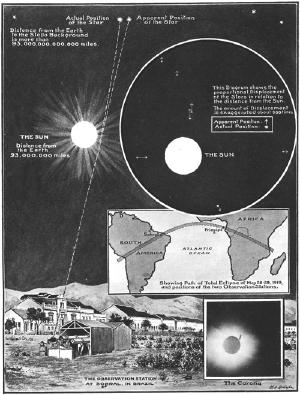
Einstein saw a parallel between the relative nature of motion in his theory of special relativity and the relative nature of gravity, and so he worked to generalize relativity to include both gravity and motion. This theory of general relativity is what he published in 1915. It was a radical proposal. In his theory Einstein argued that gravity was not a force in the way Newton had thought. Instead, gravity was an effect of a curvature of space and time. Newton had assumed space and time never changed, but Einstein proposed that space and time could be warped by large masses such as the Sun. The Sun, he argued, curved the space around it. As a result, the path of the planets curved, just as if there was a force acting on it.
General relativity explained why everything falls at the same rate, and it agreed with Newton in most cases. The downside of Einstein’s new theory was that it was very difficult to understand. Einstein had used an obscure branch of mathematics to create his theory, and few scientists were able to follow it. One scientist who was able to understand Einstein’s work was an English astronomer named Arthur Eddington.
Eddington encouraged Einstein to use general relativity to calculate the precession of Mercury’s orbit. By 1919, Einstein finished a calculation which predicted a precession of 43 arc-seconds, exactly the amount observed. While this demonstrated Einstein’s theory could be correct, it wasn’t enough. As Carl Sagan once said, “Extraordinary claims require extraordinary evidence.” and Einstein’s theory was a very extraordinary claim. Newton had worked perfectly for nearly three centuries, and unlike Einstein’s model it was beautifully simple. Newton would not be toppled by confirming an observation that was already known.
To topple Newton, Einstein would need a prediction that hadn’t been observed. One that clearly showed his theory was correct, and Newton’s was wrong. It was Eddington who devised just such an experiment. All he needed was a total solar eclipse. Newton’s and Einstein’s theories predicted almost the same results for the planets. Einstein’s theory did account for Mercury’s precession, but this was not a huge effect. Where Einstein’s theory differed significantly was in the way light behaved. If space is truly curved, then that curvature would effect light as well as planets. If a beam of light passed near the Sun, the curvature of space would bend the beam, an effect now known as gravitational lensing. If Newton was right, and space wasn’t curved, there wouldn’t be such an effect. Eddington realized that one could observe this effect during a total solar eclipse.
In 1919, Eddington traveled to the island of Principe off the coast of West Africa to photograph a total eclipse. He had taken photos of the same region of the sky sometime earlier. By comparing the eclipse photos and the earlier photos of the same sky, Eddington was able to show the apparent position of stars shifted when the Sun was near, just as Einstein had predicted.
Einstein and Eddington had proven that space and time were malleable, that they could be bent and twisted by the presence of matter, and our view of the universe was changed forever.