Post
Dark Matters
5 April 2013
Yesterday CERN (of Large Hadron Collider and Higgs boson fame) had a press release on the first results of the Alpha Magnetic Spectrometer (AMS). This led to a flurry of news articles declaring that dark matter had been directly observed. But that’s not what the results show at all.
The AMS is a particle detector located on the International Space Station, and it’s designed to detect high energy particles known as cosmic rays. Despite their name, cosmic rays are actually high energy particles, mostly protons. About 1% of them are electrons, and an even smaller percentage is the antimatter version of the electron known as positrons. These have the same mass as electrons, but a positive charge instead of a negative one, hence the name.
Cosmic rays are a challenge to observe. They have so much energy that when they strike our atmosphere they cause a cascade of particles to appear. This is useful if you want to do particle physics, but it makes it impossible to observe cosmic rays directly on Earth. Since the AMS is on the space station, it can detect cosmic rays directly. It is also the most sensitive detector of cosmic rays we have, so these first results are of great interest.
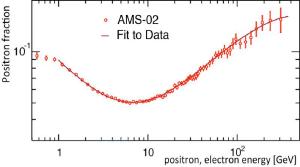
The figure here shows these first results.1 It shows the ratio of positron cosmic rays to electron cosmic rays as a function of their energy. The circles show the data with their uncertainty. What is interesting is that as you go to higher energies (to the right on the graph), the fraction of positrons increases. The reason this has hit the news is that it might be evidence of dark matter.
I’ve written about dark matter before. Basically, the idea of dark matter arose from the fact that stars in our galaxy aren’t moving at the speeds we expect. Most of the visible mass of our galaxy is near its center, so it should be the case that stars closer to the galactic have a greater speed than stars on the edge. What we actually observe is that stars on the edge have about the same speed as stars nearer the center. That means either our understanding of gravity is wrong, or there is some invisible mass (dark matter) surrounding our galaxy. Dark matter might seem the the worse of these ideas, but there is other evidence of dark matter. For example, we can see the gravitational effect of dark matter on light in some galaxies. So the evidence for dark matter is reasonably strong, and most astronomers and astrophysicists feel it’s conclusive.
The problem is we don’t know what dark matter could be. We know three basic facts about dark matter. 1) it has to have mass (otherwise it wouldn’t cause the stellar motions we observe), 2) it can’t interact strongly with light or regular matter (it makes up 95% of our galaxy’s mass, so we would have seen it by now), 3) it can’t be a type of particle we already know, such as neutrinos. Basically it has to be some new kind of Weakly Interacting Massive Particle (or WIMP). To figure out just exactly what dark matter is, we need to have some kind of direct observation of it. There are several experiments trying to do just that, and the AMS is one of them. With no evidence on the specific nature of dark matter beyond it being WIMPs, there is no shortage of ideas as to what it might be. One of the popular ideas is that dark matter is a supersymmetric particle. String theory relies on supersymmetry, which proposes that every particle has a symmetric pair (different from matter-antimatter symmetry). Thus electron - selectron, photon - photino, etc. No supersymmetric particles have been observed, but if they exist they could be a source of dark matter.
The supersymmetric dark matter model has certain predictions. One of this is that their collisions could produce an excess of positron cosmic rays at higher energies, which is what this graph shows. The line on the graph is the theoretical prediction for the simplest dark matter model, and it fits quite well. But the supersymmetric model also predicts that the fraction of positrons should drop off at higher energies which (at least yet) hasn’t been observed. There would also be a directionality to this positron bias. It should be stronger along the galactic plane (where there would be more dark matter), which also hasn’t been observed.
But saying that this data hints at dark matter is a bit disingenuous. There are lots of other sources of positron cosmic rays. Black holes, pulsars, white dwarfs, etc. These would produce similar positron distributions, and would also have a bias along the plane of our galaxy. In short, AMS has good data on cosmic rays, but nothing in the data points to dark matter more than other sources.
Unfortunately the press release for these results made the claim that these results hinted at dark matter, and could even be the first direct evidence for it. You’ll notice the graph below is fit to the dark matter model, but not any alternative model. This was done intentionally, and it helps to feed the fires of sensationalism in science reporting. Unfortunately it makes it much harder to convey what’s really going on. These results were hyped, intentionally, and they shouldn’t have been. This isn’t how science is done, and it shouldn’t be how science is conveyed to the general public.
Dark matters indeed.
Aguilar, M., et al. “First result from the Alpha Magnetic Spectrometer on the International Space Station: precision measurement of the positron fraction in primary cosmic rays of 0.5–350 GeV.” Physical Review Letters 110.14 (2013): 141102. ↩︎