Post
Dancing With
the Stars
3 April 2013
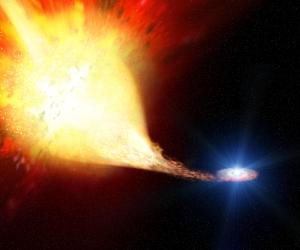
Last time I talked about how large stars can become a supernova through a collapse of their core. But this only occurs in stars much larger than our Sun. So how can a solar mass star become a supernova? For that, it has to dance with another star.
If you recall from last time, when a Sun-sized star begins to run out of hydrogen to fuse in its core, it fuses helium for a while, causing it to swell into a red giant. After this stage the star can’t fuse any higher elements, and what remains of the star compresses down to a white dwarf. All the mass of the star is squeezed to about the size of the Earth. In a white dwarf it isn’t the heat and pressure of fusion that balances against the weight of gravity, but the pressure of the electrons pushing against each other. For a single star such as our Sun, that’s the end of the story. The white dwarf simply cools over time. But for a binary star system, something more interesting can occur.
Suppose there was a binary star system, consisting of two stars similar to the Sun, and reasonably close together. These two stars will spend most of their lives as main sequence stars, but they will eventually run out of hydrogen and become red giants.
Of the two stars, one is likely to be a bit larger than the other. This means throughout it’s main sequence period the larger star will burn just a little bit hotter, and use up its hydrogen a bit faster. As a result, the larger star will enter its red giant stage while the smaller star is still a main sequence star.
A red giant is fairly large. For a Sun-like star the red giant can be as large as the Earth’s orbit. In a binary system this means the outer layers of the red giant can can be captured by the companion star. It can even engulf the two stars in what is called a common envelope. The result is that some of the mass of the larger star is transferred to the smaller star during this stage. The larger star then collapses into a white dwarf.
Some time later, the smaller star enters its red giant stage, and a similar process occurs. The smaller star swells into a large red giant, and some of the outer material is captured by the first star, which is now a white dwarf. You can see an artist’s rendering of this process in the image above.
A white dwarf is formed because the electron pressure within the white dwarf is able to balance its gravitational weight. But this electron pressure has a limit (about 40% greater than the mass of the Sun) known as the Chandrasekhar limit. As the white dwarf continues to capture mass from the other star, its mass continues to rise, approaching that ultimate limit.
As the this limit is reached, the star begins to collapse into a neutron star. This causes a cascade of rapid fusion within its core, which rips the white dwarf apart. The white dwarf explodes as a supernova. This stellar dance ends with a bang.
What makes this type of supernova particularly interesting is that it always happens with a white dwarf of about 1.4 solar masses, and it always occurs through the same general process, destroying the white dwarf completely. This means a supernova created in this way always has about the same brightness. They are called type Ia supernovae, and they are very useful to astronomers.
There are various ways to measure the distances of galaxies. One way is to observe a particular kind of star that varies in brightness, known as a Cepheid variable star. We can use these stars to measure the distance of closer galaxies, but only as far as about 90 million light years. For more distant galaxies these stars are just too dim to observe accurately. But we’ve also observed type Ia supernovae in galaxies for which we have measured their distance. We can observe how bright the supernova appear, and knowing their distance we can determine how bright they actually are. What we find is that type Ia supernova always have the same absolute brightness.
This means we can use them as a “standard candle.” If we observe a type Ia supernova in a distant galaxy, we can observe how bright it appears. Since we know how bright it actually is, we can calculate the distance to the galaxy, since the more distant a light source is, the dimmer it appears. We can therefore use this type of supernova to measure the distance to its galaxy.
Since these supernovae are very bright, we can measure the distance of galaxies billions of light years away. Without them, the size and structure of the universe would still be a great mystery.
Two stars, having a last dance, and helping us to discover the secrets of the cosmos.