Blog
Turn of the Screw
11 September 2019
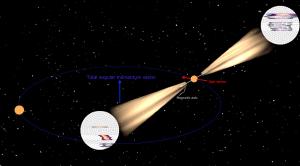
The Earth and Moon are almost a double world. The Moon is so large compared to the size of Earth that its gravitational pull affects our planet’s motion. One of these effects is known as axial precession. Just as the Moon’s pull on our oceans causes the tides to rise and fall, the Moon’s pull also tries to shift the Earth’s rotational axis. As a result, the Earth’s axis precesses over time. It’s similar to the way a spinning top slowly shifts its orientation.
We see this effect in the motion of the stars. Right now, the stars of the northern hemisphere appear to rotate about the star Polaris. We call it the north star, because Polaris is close to the north celestial pole. But this wasn’t always the case. Because of axial precession, the faint star Thuban was the north star 5,000 years ago. In about 12,000 years, the bright star Vega will be the north star.
Axial precession also occurs with binary stars. Measuring this effect is very difficult, however, because it’s often hard to measure the rotational orientation of a distant star. But we can measure the effect in binary pulsars.
A pulsar is a dense neutron star oriented in just the right way. Neutron stars have such strong magnetic fields that charged particles caught in their fields generate beams of radio light that emanate from the magnetic poles. When a neutron star is oriented just so, the radio beams sweep past the direction of Earth, creating a repeating radio pulse. We can measure these pulses to determine a pulsar’s motion and rotation very precisely. Pulsar measurements are so precise that we can use them to test general relativity. For example, observations of a binary pulsar gave us the first indirect evidence of gravitational waves.
When a pulsar’s axis precesses, the orientation of the radio beam can shift to the point where it no longer points in Earth’s direction. When that happens the pulsar goes silent. It still generates beams of radio light, but we can’t observe them.
In 2004, astronomers observed the binary pulsar PSR J1906+0746. At the time, it’s alignment was such that radio beams from both magnetic poles swept in Earth’s direction. But when it was viewed again later, only one beam was oriented toward Earth. This meant the pulsar’s orientation was precessing. So a team of astronomers gathered data on the pulsar from 2004 to 2018. They compared the data to the gravitational predictions of the precession, but with an extra twist.
The axial precession of Earth is mostly driven by Newtonian gravity. Pulsars are much more massive, so relativistic effects come into play. Einstein’s theory of general relativity predicts not only classical precession, but an extra precession that’s due entirely to relativity. The pulsar measurements are extremely precise, so the team could compare the pulsar’s precession to that predicted by general relativity. They found that observations agreed with Einstein’s theory.
These types of studies are important because they let us test the limits of general relativity. So far the theory holds up, but some future study could reveal where the theory breaks down.