Blog
Strange Light
26 October 2022
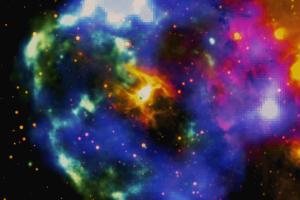
The life of every star is a fight against gravity. Stars are so massive they risk collapsing under their own weight, but this is balanced by the heat and pressure a star generates through nuclear fusion. Eventually, that comes to an end. The outer layers of a star will be cast off, and the remaining core will become a stellar remnant. Which kind of remnant depends on the mass of the core.
If the core is less than 1.4 solar masses, then it will collapse until the pressure of electrons balances its weight, thus becoming a white dwarf. If the core is more massive than that, up to perhaps 3 solar masses, it collapses until neutron pressure resists, creating a neutron star. Beyond that, the core will collapse into a stellar-mass black hole.
At least that seems to be the case. The most massive white dwarf we’ve found is about 1.35 solar masses, and the smallest black hole we’ve observed is about 2.6 solar masses. The most massive confirmed neutron star is about 2.14 solar masses. Of course, the cut-off ranges aren’t absolute. A stellar core might be a bit under 1.4 solar masses but experiences an explosive collapse that pushes it to become a neutron star. Neutron stars of 1.2 or 1.3 solar masses would be unusual, but not impossible. But recent observations of the neutron star HESS J1731-347 seem to give it a mass of 0.77 solar masses, which shouldn’t be possible.1
Neutron stars are notoriously difficult to mass. They are only about 20 kilometers in diameter and can be seen by the x-rays they give off. To calculate the mass you generally either need the neutron star to be a companion of a star, so that you can determine mass by orbital dynamics, or it needs to be a pulsar so that you can use radio observations to get a mass estimate. HESS J1731-347 is neither of these, but it does have a remnant nebula surrounding it. That remnant is illuminated by a nearby regular star, which we do know the distance of. The regular star was mapped by the Gaia spacecraft, so we know its distance really well. From that data, the team looked at the reflected light of the nebula to determine the distance of HESS J1731-347. It’s about 8,000 light-years away, which is closer than we thought.
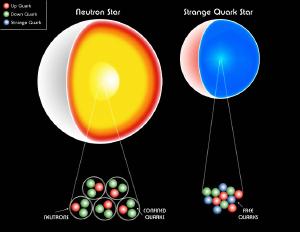
Knowing the distance, the team then looked at x-ray observations of the neutron star. Given their luminosity, the calculated mass comes out to be 0.6 - 0.9 solar masses, which is far below the white dwarf mass limit. If this mass is accurate, our understanding of neutron stars is off. One possibility is that we don’t understand how neutron stars form. The authors propose another possibility, which is that it could be a type of quark star known as a strange star.
The standard view of neutron stars is that they are mostly neutrons. Some theorists argue that within the core the boundary of neutrons might break down, creating a soup of up and down quarks, thus a quark star. A strange star would be one where colliding quarks in the core create strange quarks, so that the neutron star has a core of up, down, and strange quarks. All of this is hypothetical, but since strange quarks are twenty times more massive than up and down quarks, a strange star would have a much greater density than a normal neutron star. Thus, it could hold itself together even with a mass much smaller than the Sun.
The third option is, of course, that the mass result is wrong. This is only one result, and several factors could make the neutron star appear dimmer than it actually is, thus skewing the mass result. It’s an interesting result, but not conclusive. Even the authors acknowledge that more observations are needed to confirm the result. It is indeed a strange sight, but it might not be a strange star.
Doroshenko, Victor, et al. “A strangely light neutron star within a supernova remnant.” Nature Astronomy (2022): 1-8. ↩︎