Blog
Unscrambling a
Quantum Egg
15 March 2019
The laws of thermodynamics are deeply rooted into the fabric of reality in ways we still don’t fully understand. At the same time, the basic principles of thermodynamics are so much a part of our lives we understand them intuitively. We all know that a hot cup of coffee cools when left sitting on the kitchen table, or that a dropped dish can be mended but not unbroken. Or, as my Grandfather used to say, “you can’t unscramble an egg.”
This idea stems from the Second Law of Thermodynamics, which states that the entropy of a system can never decrease. There’s several ways to quantify entropy, but one way is to say it is a measure of the disorder of a system. According to the second law, a hot cup of coffee has relatively low entropy because its heat is constrained to the cup. Over time the heat spreads into the table and surrounding air, since spread out heat has higher entropy. Thus hot coffee grows cold. A plate has low entropy because it was made to have a clear structure. Shatter the plate and the pieces fly apart, increasing entropy.
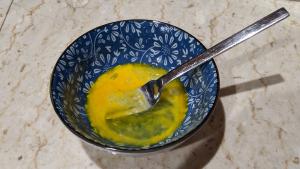
Of course this concept of entropy is a bit simplistic. To say that one thing is more disordered than another is vague, and entropy is a statistical property, not an absolute one. Consider the motion of air in a room. All the air molecules bounce around and against each other, and this tends to mix them up. If someone were place a cup of coffee on the table, it wouldn’t be too long before the aroma would spread to fill the entire room. The random motions of molecules spreads the smell of coffee over time. But random motions can also be felicitous. There is a chance that the aroma would drift only to your nose, or only to a single corner of the room. The chance is astronomically unlikely, but not zero. In other words, it is hypothetically possible to unscramble an egg, but the odds are so long it might as well be impossible.
It is possible to create a system where entropy decreases for a short time.1 Gaming the system, as it were. The entropy quickly goes back to increasing again, so these experiments are interesting but unexpected. This is exactly what thermodynamics predicts. But what happens when you aren’t talking about eggs and rooms full of air, but really small things where quantum rules come into play? That’s where things get a bit more interesting.
Despite all the odd behavior of quantum things, our basic intuition still applies when it comes to entropy. There’s a fuzzy behavior to quantum objects, so instead of describing where it is at any given time, we can only describe where it is likely to be found.2 Like a game of hide and seek, until we actually find it, we only know if we’re getting warmer or colder. Despite this difference, the location of a quantum object is similar to a cup of hot coffee. If the object is known to be somewhere in a small region initially, over time its location becomes more diffuse and uncertain. Just as the heat of the coffee diffuses over time, so too does the likely location of a quantum object. The difference is that the diffusion of heat is caused by random chance, whereas the quantum effect isn’t, so it wasn’t clear whether you could game a quantum system to reverse entropy. But a recent experiment3 has found that it can be done.
Unfortunately, most of the popular press on this work has described it as a breakthrough where “scientists reverse time,” as if they’ve created some kind of time machine in the lab. It doesn’t help that the paper itself talks about the “arrow of time and its reversal.” I’m not keen on the authors’ use of the phrase “time reversal,” but the work does have implications about the arrow of time.
Most of us think of time as a shift from past to present to future. Time flows, and we are dragged along with it. But in physics there’s no obvious reason why time behaves that way. Time doesn’t have an inherent direction in physics. Suppose I were to show you two videos where a billiard ball bounces against the side of a pool table. If I told you one of the videos was reversed, could you pick which one? Probably not. On the other hand, if I showed you a video of a plate shattering, and another of a plate unshattering, you’d easily know the latter was reversed. Plates don’t spontaneously assemble themselves. In other words, the increasing disorder of the plate tells you the proper direction of the video.
In physics we call this the arrow of time. It means that the direction of increasing entropy tells us the direction that time flows. By decreasing the entropy of the quantum system, the team “reversed” the arrow of time. But they did not actually reverse time any more than if they had built a mechanical clock that runs backward.
Andrieux, D., et al. “Entropy production and time asymmetry in nonequilibrium fluctuations,” Phys. Rev. Lett. 98, 150601 (2007). ↩︎
This is known as the wavefunction, or quantum state of the object. ↩︎
G. B. Lesovik, et al. “Arrow of time and its reversal on the IBM quantum computer,” Scientific Reports, 9, 4396 (2019). ↩︎