Blog
The Great Migration
4 February 2014
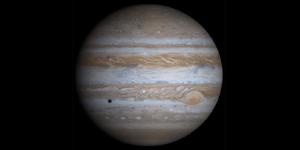
We know that our solar system formed during the Sun’s youth, when it still had a protoplanetary disk. Such a disk contains gas and dust left over from the star’s formation, and over time the planets coalesced from that material. We can see such protoplanetary disks today around young stars, such as those forming in the Orion nebula. You might think that the planets formed within the planetary disk basically in the region where they are today, but in fact the dynamics of a protoplanetary disk are very complex, and we have a great deal of evidence that the planets in our solar system migrated over time to their current positions.
Through computer simulations we know that early planets migrate following some general trends. The reason why this early period is so difficult to understand is that a protoplanetary disk is basically a fluid of gas and dust. Because the gas is typically ionized, it interacts with the magnetic field of the central star. Because of the dust collisions and clumping, there is also turbulence within the disk. In physics, such a system can be described by magnetohydrodynamics, but the equations for such a system are extremely difficult to analyze. Even simple models are computationally intensive. Still, with modern supercomputers we have been able to discover some general trends.
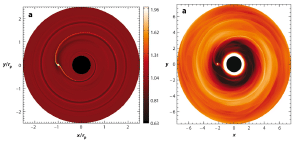
Low mass planets (less than 10 Earth masses) don’t strongly disturb the overall structure of the protoplanetary disk. Their interactions with the disk induce what is known as a spiral density wave within the disk. One wave spirals inward in the leading direction of the planet’s motion, while another wave spirals outward on the trailing edge. Since the drag from the outer spiral is typically larger than the drag from the inner spiral, the planet will tend to move closer to the star fairly quickly. This is known as Type I migration. For high mass planets (greater than 10 Earth masses) not only is a density wave induced, but the planet creates a gap in the protoplanetary disk. You can see this in the right figure above. This means that while there is still a net inward drag, it is smaller. So the planet would gradually move inward during its formation. This i known as Type II migration. The net effect of both of these dynamics is that planets formed in the disk will tend to move toward the star. This may explain why many Jupiter-type planets are found so close to their stars.
Our own solar system is a bit unusual in that the largest planets, Jupiter and Saturn, are far away from the Sun. This is one clue that the outer planets made an outward migration. This is where things get tricky, because it is likely due to strong interactions between the large planets.
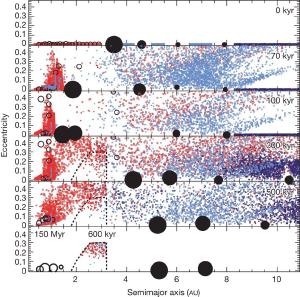
One of the ideas is that as Jupiter was forming it moved much closer to the Sun. It then entered a gravitational resonance with Saturn, where Jupiter would make 3 orbits for every 2 of Saturn. This 2:3 resonance would then drive the planets outward. Subsequent interactions with Uranus and Neptune would drive those planets outward as well. This model, known as the Grand Tack, could explain why Mars is relatively small for a terrestrial planet, as well as why the asteroid belt region did not form a planet.1
Another model, known as the Nice (pronounced neese) model, posits that Jupiter was roughly at its current distance when it entered a 1:2 resonance with Saturn. The resulting resonance drove Neptune (initially closer than Uranus) to the outer edge of the solar system, pushed Uranus and Saturn outward, and scattered much of the remaining protoplanetary material out to the farthest reaches of the solar system. This model explains much of the features we see in the modern solar system. It could also explain the Oort cloud and the late heavy bombardment period.
There is still a lot we don’t know. As we learn more about other planetary systems, as well as more about our own solar system, we will surely learn whether either of these models are correct, or neither, or a combination of both.
Walsh, Kevin J., et al. “A low mass for Mars from Jupiter’s early gas-driven migration.” Nature 475.7355 (2011): 206-209. ↩︎