Blog
Gravitational Constant
23 March 2014
One of the big criticisms of astrophysics is how it claims to actually know what has occurred billions of light years away, and thus billions of years ago. After all, no human was there, so who’s to say that the physics we have on Earth is the same as the physics of the distant cosmos? It’s a valid criticism. Astrophysics works with the assumption that the laws of physics are the same everywhere. That’s a huge assumption given that our most distant space probe has barely left the solar system. So how do we know our assumption is valid?
It turns out there is a way to test this assumption, and that is by looking at what are known as physical constants. Within physics there are certain things such as the speed of light and the charge of an electron that always have the same value. They are known as physical constants, and if the laws of physics are the same everywhere then these values should never, ever change.
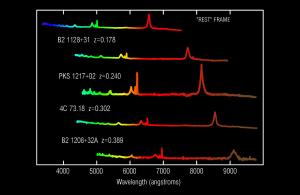
If any of these constants did change, then it would affect what we observe. For example, if the speed of light changed, then the pattern of line spectra from different elements would also change. Not just redshift or blueshift, but the spacing between lines would shift. So when we look at distant objects in the Universe, we can measure whether these types of relations change over time.
Several of these types of experiments have been done, and so far all of them have found absolutely no change to within the limits of the experiment. We know, for example, that the ratio of proton mass to electron mass is has changed by no more than 1 part in a billion over the past 7 billion years. We know the speed of light has remained constant for at least a similar period.
Now a new paper in the Publications of the Astronomical Society of Australia adds a new constant to this list.1 The universal constant of gravity, known as G. The gravitational constant G is what determines the strength of gravitational attraction between two masses. If G were larger, then masses would be attracted more strongly.
What the authors of this paper did was to look at 581 type Ia supernovae. This particular type of supernova has a consistent absolute magnitude (brightness), which is why they are used as standard candles to measure galactic distances. They can be identified by the way they dim over time, which is due to the radioactive decay of elements such as Nickel-56. If the gravitational constant changed over time, the absolute magnitude of these supernova would change relative to the decay of Nickel-56. What they found was that there was no observable difference in these supernovae. This means that G has changed by no more than one part in a hundred million over the last 9 billion years.
Once again, the physics of the Universe appears to be universal. To the limits of our observations, physics is the same everywhere and everywhen. So we really can have confidence that what we observe “out there” can be interpreted by our understanding of physics here on Earth.
Mould, Jeremy, and Syed A. Uddin. “Constraining a possible variation of G with Type Ia supernovae.” Publications of the Astronomical Society of Australia 31 (2014). ↩︎